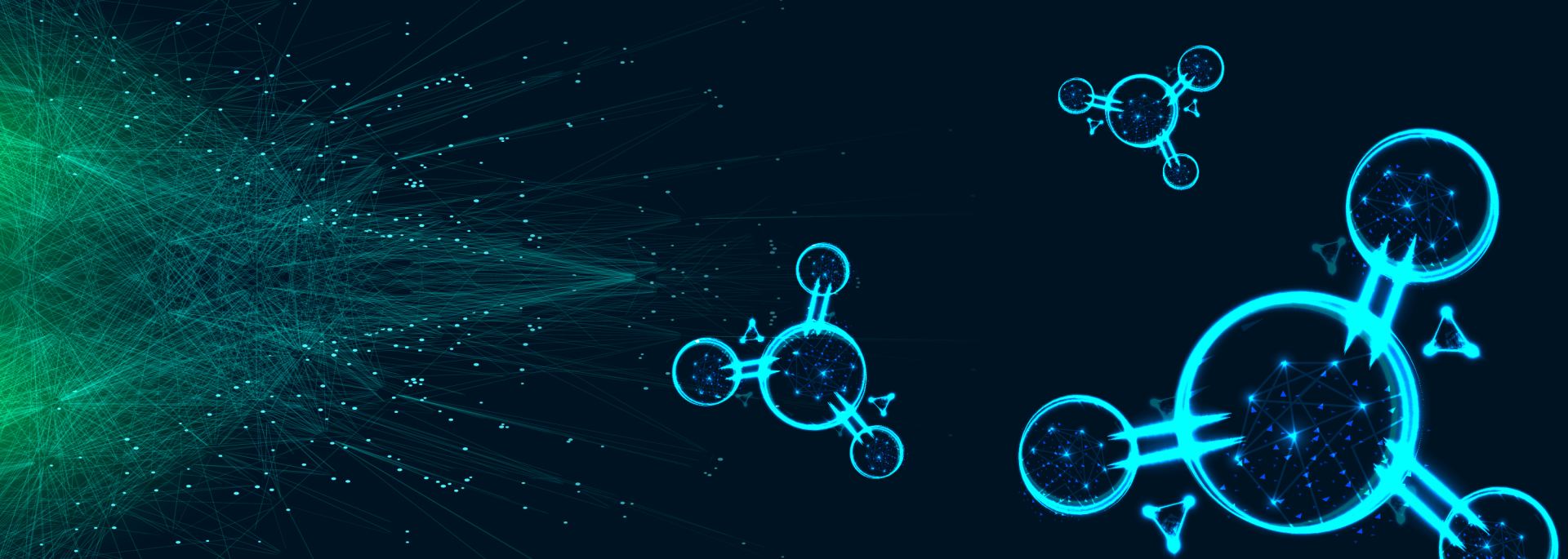
Team Devises Crystal Clear Solution to Quantum Computing Puzzle
University of Texas at Dallas researchers have developed a new approach that addresses challenges in the field of quantum computing and has the potential to revolutionize computing, communications and electronic security.
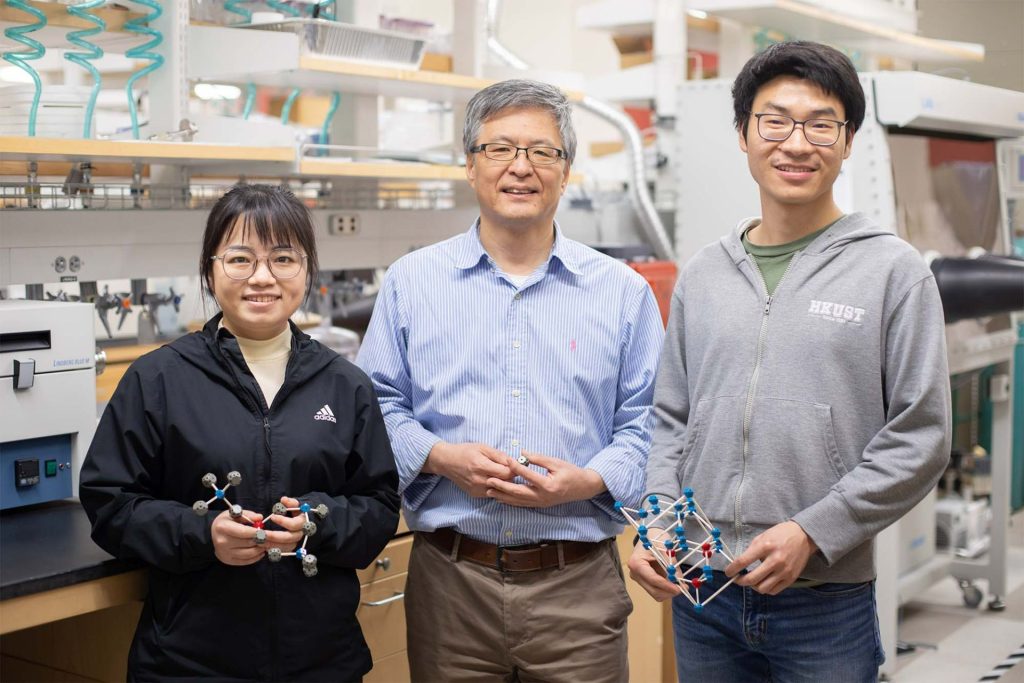
From left: Xiuyao Lang, Dr. Kyeongjae Cho and Yaoqiao Hu found that making quantum computing qubits from thin 2D sheets of crystals called transition metal dichalcogenides (TMDs) can enable researchers to avoid the challenges of using synthetic diamonds. The researchers are holding atomic structure models of solids, with the white sticks representing the bonding between atoms and the blue, red and gray balls representing different atoms in solid structures.
University of Texas at Dallas researchers have developed a new approach that addresses challenges in the field of quantum computing and has the potential to revolutionize computing, communications and electronic security.
To make solid-state qubits, the basic information unit for quantum computers, a defect must be inserted into the solid material to control the spin states of electrons. Creating and positioning the defect, however, especially in the most commonly used solid material — synthetic diamonds — poses a major challenge.
UT Dallas researchers found that making qubits from thin, two-dimensional sheets of crystals called transition metal dichalcogenides (TMDs) instead of synthetic diamonds can solve this problem. Led by Dr. Kyeongjae Cho, professor of materials science and engineering in the Erik Jonsson School of Engineering and Computer Science, the researchers published their findings online Dec. 6, 2022, in Nature Communications.
“When you are sitting on the tarmac during the summer in Dallas, it can be really hard and inefficient to transfer all the heat generated inside the aircraft to the surrounding outdoor environment,” he said. “The same is true if a military aircraft is flying very fast at low altitude in a hot climate.”
Cho said the ability to control a qubit’s properties allows researchers to develop a better way of maintaining the qubit conditions needed for two essential processes: entanglement and long-distance communication over fiber optic cable networks.
“While diamond qubits must first be converted to the
correct infrared wavelength, TMD-based qubits do
not need to be converted.”Dr. Kyeongjae Cho
professor of materials science and engineering in the Erik Jonsson School of Engineering and Computer Science
Quantum entanglement is a phenomenon in which qubits are linked and can transmit information to one another regardless of their physical distance. It’s the underlying process that enables quantum computers to process huge amounts of information simultaneously. But entanglement can only be maintained under precisely controlled conditions. Optimizing qubit properties using TMDs is a key step in this process and should help produce reliable quantum information processing and communication, Cho said.
TMD qubits provide another advantage over those made from diamond. Long-distance communication between computers takes place over fiber optic networks and requires the signals to be in the form of infrared light.
“While diamond qubits must first be converted to the correct infrared wavelength, TMD-based qubits do not need to be converted,” Cho said.
UT Dallas researchers also collaborated with researchers from the Massachusetts Institute of Technology and other institutions to develop a method for fabricating smaller 2D TMD single-crystal materials by geometric confinement. That research was published Jan. 18, 2023, in nature.
Yaoqiao Hu, a materials science and engineering doctoral student at UT Dallas and a co-author of both papers, became interested in the research while working on Cho’s quantum computer materials project.
“I think quantum computing is very interesting — it can solve problems that our classical computers cannot solve,” Hu said. “I want to use materials science to provide technology and make a contribution to the platform to achieve quantum computing.”
Hu completed an internship at Texas Instruments last summer, and he expects to continue his work at the company after he finishes the last year of his doctoral program.
Xiuyao Lang, also a materials science and engineering doctoral student and co-author of the Nature Communications paper, enrolled at UT Dallas in 2021 to study quantum technology.
“I think it’s a very good opportunity for me to learn about quantum computing,” said Lang, a Eugene McDermott Graduate Fellow. “It’s a good start for my doctoral research.”
Additional UTD co-authors of the Nature Communications paper article include lead author Yeonghun Lee PhD’21, now an assistant professor of electronics engineering at Incheon National University in South Korea, and Dr. Dongwook Kim, a former postdoctoral researcher in Cho’s lab. Researchers at the University of California, Santa Cruz and the University of Washington also contributed.
The UT Dallas researchers were supported by the Applications and Systems-Driven Center for Energy-Efficient Integrated NanoTechnologies, a component of the Defense Advanced Research Projects Agency’s Joint University Microelectronics Program. Additional support came from the UTD Center for Atomically Precise Fabrication of Solid-State Quantum Devices, the UTD Seed Program for Interdisciplinary Research (SPIRe) and the National Research Foundation of Korea. Researchers from collaborating institutions received support from the Army Research Office and the National Science Foundation (NSF).